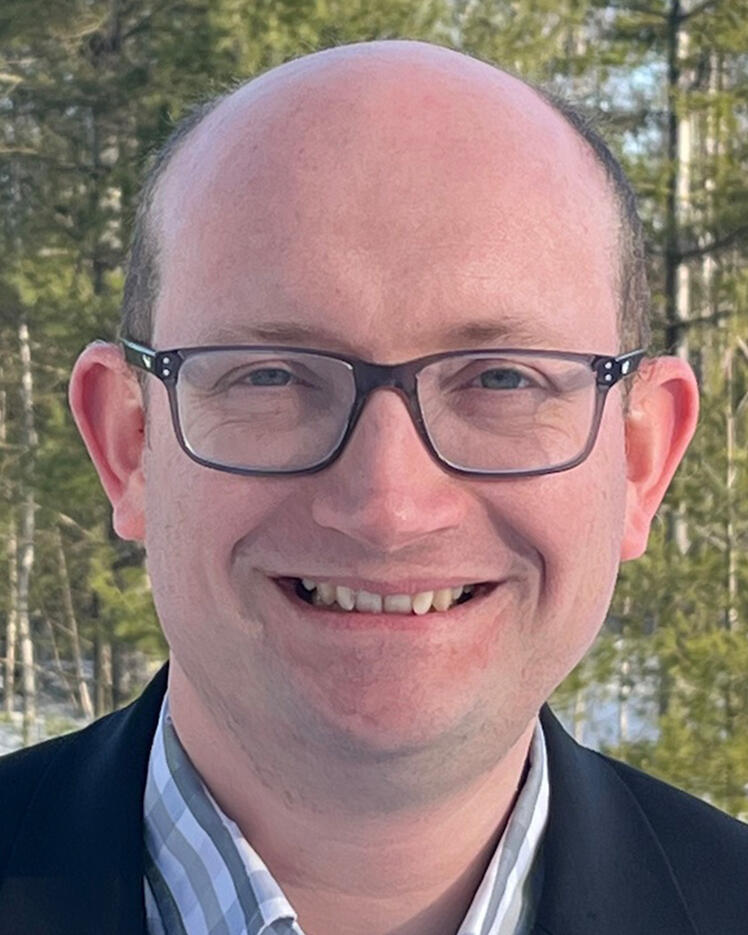
Paul François
Biography
Paul François is a full professor in the Department of Biochemistry and Molecular Medicine in the Faculty of Medicine, Université de Montréal, and an adjunct professor in the Department of Physics at McGill University.
François is a biophysicist whose research focuses on the application of computational methods (including machine learning) to evolution, embryonic development and immunology. He is an associate academic member of Mila – Quebec Artificial Intelligence Institute.
Past positions:
- Associate professor of physics, McGill University (2016–2023)
- Assistant professor of physics, McGill University (2010–2016)
Education and training:
- Postdoc, Siggia Lab, The Rockefeller University, U.S. (2005–2010)
- PhD in theoretical physics, Hakim Lab, École Normale Supérieure / Université Paris VII, France (2002–2005)
- MSc in theoretical physics, École Normale Supérieure / École Polytechnique, France (2001–2002)
- BEng, major in physics, École Polytechnique (1998–2001), Promotion X 98
Some awards:
- 2019 Rutherford Memorial Medal in Physics, Royal Society of Canada
- 2017 CAP Herzberg Medal, Canadian Association of Physicists
- 2015 McGill Principal’s Prize for Outstanding Emerging Researcher
- 2014 Simons Investigator in Mathematical Modeling of Living Systems
- 2007 Lavoisier Postdoctoral Fellowship (from France’s Ministry of Foreign Affairs)
- 2007 Prix Le Monde de la recherche universitaire (awarded by the French newspaper Le Monde)